by Jeffrey S. Hansen
To state the obvious, more big-rig (Class 8) trucks are on the road than ever before. A freeway trip at two a.m. in the morning is telling: Trucks are spaced less than a quarter of a mile apart when only a few years ago, at the same early hour, a rural night would have been nearly dark to the horizon. Simultaneously, truck stops are jammed with trucks and their sleeping drivers, as are rest areas from I-5 to I-95 and I-10 to I-90. In addition, every available yard of the approaches and exits to and from these rest areas glow with cab and trailer marker lights. By dawn or earlier, the trucks are on the road, competing with commuters and other motorists. While an extra lane might alleviate chronic bottle necks, the low-hanging fruit of an easy freeway engineering solution has long been harvested, especially in regions that are cursed with the nastiest aspects of traffic congestion.*
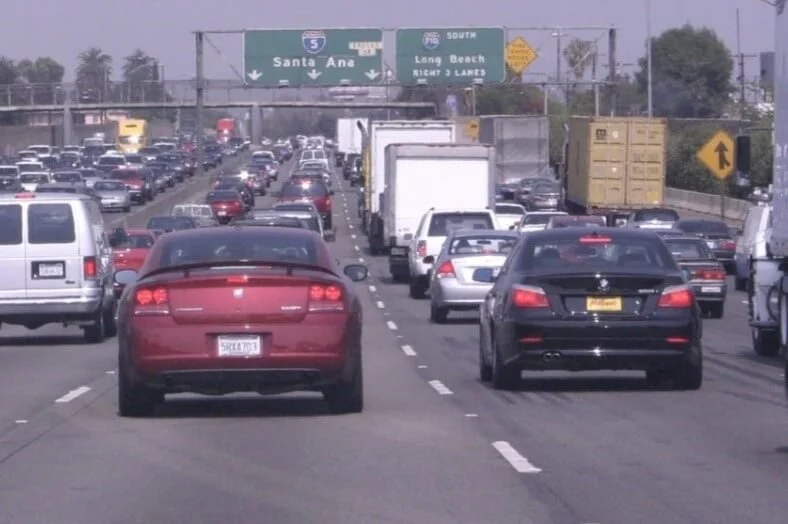
In contrast, railroads are relatively calm, a result precipitated by changing shipping markets, along with technological improvements. Coal shipments, for instance, have weakened substantially and are likely to continue sliding downward due to environmental concerns as well as economic realities (i.e. electric utilities are substituting inexpensive natural gas for coal). Crude oil shipments from new and revitalized plays may likewise decline as average vehicular fuel efficiencies continue to improve. Should personal electric vehicles become omnipresent, as many analysts have projected, the use of gasoline will ebb at a quickening pace, and the demise of much of the petroleum industry will inevitably follow. Other bulk shipments, such as fertilizer, will soften as farmers learn to eliminate wastage by employing new technologies that allow them to pinpoint right-sized applications. Even the demand for lumber could conceivably dwindle as timber resources wane, younger generations become accustomed to smaller urban dwellings, and technology renders the common 2 x 6 obsolete. Conversely, the shipment by rail of manufactured products over medium-to-long distances may grow, thanks largely to containerization and intermodal logistics.
Economically, shipping by rail is two to four times less costly than shipping by road. A locomotive engineer and conductor can move a hundred-car train of merchandises that would require more than two hundred teamsters, if the goods were instead delivered by truck. The coefficient of rolling resistance of a truck tire on asphalt is three to four times greater than the coefficient of rolling resistance of a steel wheel on a steel rail. With notable exceptions, railroads generally are free of unexpected traffic congestion. Accidents involving trains occur less frequently than trucking accidents and result in far fewer injuries, regardless of the criterion employed. The operating ratio of a trucking company is typically 95 percent while that of a railroad is currently between 60 and 70 percent and may be headed even lower. Environmentally, the transport of goods by rail produces fewer pollutants and greenhouse gasses than nearly any other means of carriage. (Ships, barges, and pipelines may be possible domestic shipping alternatives, but characteristically, these modes are applicable and practical for only a narrow range of bulk commodities.)
Why then, if railroads seem to promise superior economies and other advantages, would a shipper, apart from bulk commodity producers, prefer to ship by truck? Several reasons easily come to mind: Roads go everywhere; tracks do not. Trucking has multiple, direct-route choices, while railroad routes are geographically limited. Trucks seldom sit for extended spells in sidings or linger in marshalling yards. A loaded truck typically has one destination, while a train with multiple carloads, each having its own disparate destination, requires multiple sortings. Trucks are effectively on call, while railcars are not as readily summoned. And, on average, trains are slow.
If one word could sum up the singular advantage of shipping by truck, that word would be “EXPEDIENCY”, the quality of being best suited to the end in view.
Does a lack of superior expediency mean that railroads are forever limited, notwithstanding the long and impressive list of relatively recent technological railroad advancements? (Indeed, that list includes containerization; intermodality; double stacking; distributed power; centralized signaling; computerized, GPS-aided shipment tracking; computerized scheduling; high-mileage, low-maintenance AC locomotives; radial, high-adhesion locomotive trucks; concrete ties; welded rail; and longer trains.)
With each hard-won advancement in RR tech., it must be maddening for railroaders, while observing their trucking brethren spend so little effort in comparison, to continually discover that they will be at best oh so-close but never equal to their highway competitors in terms of shipping expediency.
How can railroads conceivably overcome the greater expediency of trucks? How can a railroad become competitive in short-haul markets? If it is thought essential to get trucks off the roads to avoid a hurry-up-and-wait future for growing populations, how can railroads be a part of the solution? And, lest they forget their responsibilities to progress environmentally, how can railroads move beyond Tier 4 -- the latest and most challenging standard applicable to diesel engines -- and lower their carbon footprints?
The following look ahead advocates that the path forward must embrace an enhanced system of intermodality to achieve a conveyor belt-like railroading/trucking partnership that never stalls or stops. Railroads, in addition, must be electrified.
Readers likely will surmise that such a system will be horribly expensive and that the effort might take so long to implement that the outcome could simply become a short-lived and futile technological advancement, like the Erie canal or the pony express. Agreed, considerable risk exists, but apprehensions should not quell at least a preliminary exploration. Fortunately, modeling, and other means of virtual analysis, particularly of economic feasibility, are available to identify and perhaps ameliorate perceived misgivings.
Visualization of a Plan: A Hypothetical Example
Workers are loading a 53-foot container at a manufacturing plant -- its shipment is destined for a large retailer’s distribution center that is 250 miles from the plant. Previously, the empty container had been delivered to the manufacturer’s dock by a truck, positioned within a jacking apparatus adjacent to the dock, and automatically lifted a few inches vertically to clear the truck trailer. The truck and trailer then departed to another assignment, and the container was again vertically and laterally repositioned a few inches to align the container for loading.
When loading is completed, the plant shipping department will call for a truck to transport the container to a railroad freight depot that is five miles away. Like a taxi for hire, the truck will arrive at the plant within minutes. Its spine-like trailer has been designed specifically for container pickups. The truck itself weighs significantly less than other Class 8 trucks, allowing for a greater payload. It will seldom venture further than 50 miles from its base, never see steep grades, or likely reach a speed greater than 60 mph. The taxi-truck driver typically works an eight-hour shift and has no need for a sleeper cab. The driver spends most nights at home, and lives within a short commute to his or her employer. Eventually, taxi trucks will be fully automated and require no drivers.
Upon arrival at the manufacturing plant, the taxi-truck driver, guided by sensors and lasers, backs the truck’s trailer under the loaded container. When the trailer is situated beneath the container, additional sensors and mechanical movers adjust the jacking apparatus to locate the container precisely in line over the trailer.
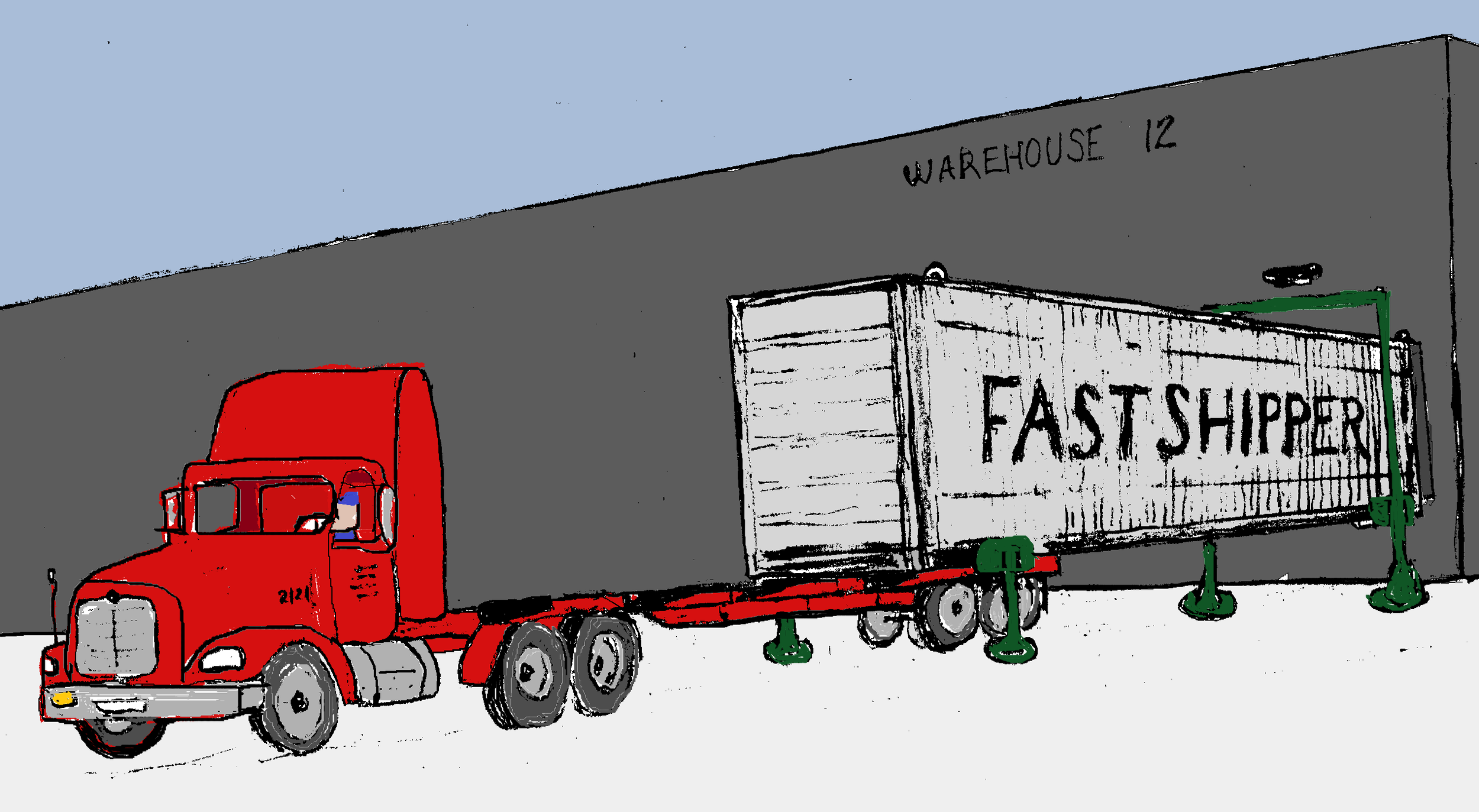
The container is then lowered to engage locating pins on the frame of the trailer. Once seated, it is automatically locked in place. After sensors inspect and certify the stability of the load and vehicle, the driver is notified to proceed. Departure time and date information are added to a shipment database that is immediately available on a shipping web site. The pickup has been accomplished in three to four minutes. The quickest route to the nearest railroad freight depot, taking into account traffic, road construction, and weather conditions, is computed and relayed to the taxi-truck driver. Compensation for the trip will be electronically credited to the truck owner’s bank account when the load has been delivered to the freight depot.
The railroad freight depot is a small facility, (although depots are not size limited) and contains an openair structure that encompasses a road-to-rail-to-road container bridge crane. The depot is one of three that are spaced along a 100-mile north-south legacy branch rail line that was first dedicated in 1910. The branch connects with the east-west mainline of a Class I railroad. The branch line, as well as the Class I, are electrified and double tracked for opposed unidirectional running.
The transfer bridge at the depot is equipped with a fixed overhead loading/unloading apparatus (called a LULA) for transferring containers to and from railroad well cars and taxi trucks. The LULA apparatus is sited above the siding tracks and the taxi-truck access road.

When the taxi truck arrives at the bridge crane, its container is detached from the trailer, lifted a few inches, and moved laterally to a precise position over a waiting railroad well car. The container is lowered, and secured in place in the well car, all without a human operator. Having accomplished this segment of the container’s journey, the taxi-truck driver may exit to a nearby holding area and wait for another incoming container that will soon arrive at the depot via another well car. Alternatively, the driver may leave the depot for a container pickup elsewhere.
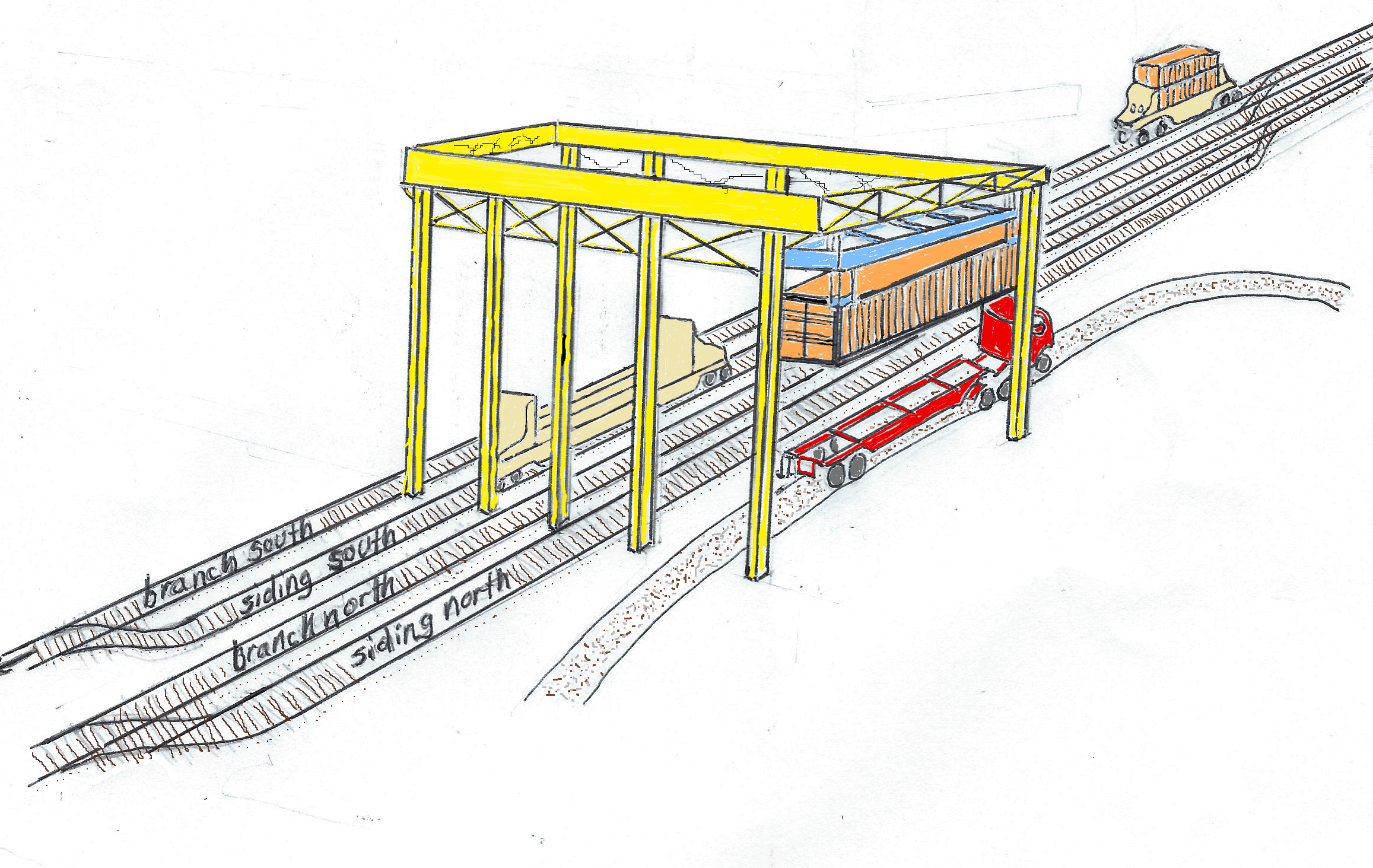
Each well car is designed to operate autonomously, like a truck on rails or a rail-diesel-car (RDC), minus an engineer. Its electric motors are included within its bogies. (The term “bogie”, synonymous with the term “truck” in American railroad-car parlance, is used here to avoid confusion with referrals to highway “trucks” that appear elsewhere in the narrative.) The well car has no couplers, but instead has a pair of European-style spring-loaded buffers on each end. It utilizes virtual coupling to form temporary multiple well-car groups, referred to as platoons. Virtual coupling, in which well cars are spaced inches apart without physical contact, is achieved through technologies such as positive train control, vehicle-to-vehicle (V2V) communication, and Light Detection and Ranging (LIDAR). The depressed well design allows the double stacking of containers.
The well car with its container departs the depot under computer control and is switched to a south branch line automatically. If a multi-well-car platoon is traveling the branch near the depot, both the platoon and the departing well car will adjust their speeds to allow the departing well car to attach itself virtually to the front or rear of the platoon. The new multiple-car platoon proceeds along the branch to its juncture with the east-west main line. The use of multiple-car platoons reduces aerodynamic drag and simplifies congestion issues that may arise at rail and road intersections.
In other instances, the controlling dispatch computer may decide that it is advantageous to insert the departing car into a slot within a multiple-car platoon that is approaching the freight depot. The slot is created by severing the virtual coupling and accelerating the leading cars ahead of the desired slot while decelerating the trailing cars. In this case, the car that is departing the depot is momentarily retained on the depot siding track until the leading half of the platoon on the branch line has passed the depot siding switch, thereby exposing the slot. The siding switch is rapidly aligned to the depot siding track, and the departing car exits to the branch line. After the departing car has completed its entry into the slot, the switch is realigned just as rapidly to close the branch line, thus enabling the trailing cars, which are now behind the inserted car, to speed up and, once again, complete the platoon. The platoon may need to be gapped one or more times to allow additional car insertions at other freight depots that service the branch along the way.
A loaded well car that is traveling within a multiple-car platoon also may be scheduled to deliver a container to a depot. To accomplish the exit of the well car from the platoon, gaps before and after the departing car are opened, and the steps comprising car insertions are similarly executed in reverse order. Switching yards are eliminated, and well cars are kept moving. The movement of container-carrying well cars entering and leaving branch and main lines is analogous to the merging and exiting of trucks by means of on and off-ramps at freeway interchanges.
At the end of its journey, the well car with its 53 ft container exits the mainline to a dedicated spur that services the large retailer’s distribution center to which it has been directed. The exit is undertaken utilizing the same operating procedure just described for extracting cars at a freight depot. When the delivery of the container to the retailer’s dock is completed, the well car is dispatched to another shipper or to a freight depot for immediate disposition or temporary storage.
If the retailer’s distribution center had not been located on a spur, a taxi truck would have been summoned to coincide with the arrival of the well car with its container at a freight depot near the center. The container would be transferred to the taxi truck by a LULA, the taxi-truck would transport the container to the distribution center, and the container would be unloaded at the mechanized dock of the center.
An Examination of the Components
Many of the components described in the visualization do not yet exist. Some components may have whole or partial precedents, while others will require significant research and development. R & D may reveal a need for substantial alterations or suggest, after a subsequent analysis of technical and/or economic findings, that a component be abandoned for an alternative.
Well Cars and Containers
Prevalent on nearly all railroads, well cars are necessary in order to allow the double stacking of containers. To facilitate double staking, the integrated frame of a well car is dipped between the car’s two bogies to reduce the overall height of a load and lower the center of gravity. In total, double stacking can double the capacity of a railroad. Since a beefed-up structure is not required to transfer tensile forces from a locomotive to a sting of cars in tow, well-cars for the envisioned railway could be redesigned and simplified. Frames could be constructed from fiber-reinforced composites or other high strength-to-weight materials to reduce transport energy needs and increase payloads. Initially, existing well cars might merely be modified to operate on the proposed railroad.
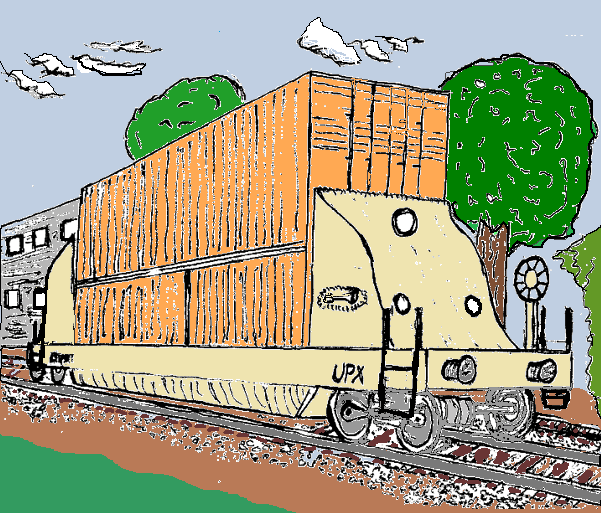
Each well-car bogie will be powered by one or more electric motors. Differentials would allow the inner and outer wheels on the radii of track curves to rotate independently as the well car negotiates the curves. Wheel/rail adhesion will vary in direct proportion with the weight of a container load, but should be adequate for all rail, weather, and grade conditions negating a need for sanding. Electrically actuated disk brakes will replace air brakes and be fully applied when not charged, and fully released at a maximum charge. Brakes will be engaged when the car is at rest.
Bogie performance will be monitored by sensors that note critical temperatures, vibrations, and electrical resistances. With a single point of connection to a well car frame, bogies can be quickly exchanged to accomplish scheduled maintenance or unscheduled repairs. Bogie replacement might be completed expeditiously at freight depots that include a small facility for the purpose.
Bulkheads at each end of a well car will be equipped with head and tail lights, a horn, and a bell or other warning device and will be streamlined to reduce air resistance. The well-car frame will not have knuckle couplers but rather will support buffers and employ virtual coupling to loosely link cars within multiple car units to enable platooning. The close distances between the cars comprising a platoon will be maintained by utilizing electronic means to accelerate and decelerate the cars simultaneously. Platooning will promote greater fuel economy due to reduced air resistance, and decrease congestion at road intersections.
Shipping containers will have standardized dimensions. While most containers will be used for boxed or palletized freight, container variations may include refrigeration units that automatically plug into wellcar electrical connections; open and closed-top hoppers for grain, fertilizers, rock, and other bulk commodities; and tanks within frameworks for liquid and gaseous fuels and chemicals. Grain containers might be loaded at farms, transported by taxi trucks to rail depots, transferred to well cars, and moved by rail to domestic customers or deep-water shipping docks. Grain could be temporarily stored in the containers, possibly eliminating redundant silos. The containers could be made of lightweight materials.
People pods will have the footprint of a container and will be fitted with seats, windows, interior lighting, and HVAC systems. The people pods will be used to transport passengers over short to medium distances. For instance, airline passengers, who reside in rural towns that are, say, 100 miles from an urban airport, could board the pod close to home. A taxi truck would be employed to drive the pod to a nearby railroad freight depot where it can be transferred to a well car and immediately dispatched to a depot that is at or near the airport. Passengers at rail-served airport depots might exit directly to a terminal or remain in the pod for a short taxi-truck ride to the terminal.
Taxi Trucks
Conceptually, taxi-truck service for container transfers between railroad depots and customer loading docks will be similar to automotive passenger taxi-fleet services. Some taxi trucks will be on call while others will wait at railroad freight depots and be immediately ready for container transfers when well cars arrive. While meeting Class 8 requirements, the trucks could be designed specifically for short, local hauls and would not need amenities such as sleepers. Truck designs could be customized with regard to area topographies, climates, and operating conditions in order to maximize fuel economy. Some taxi trucks could be fitted with electrical connections for container refrigeration units or people-pod accessories. At a future date, the taxi trucks will be driverless and electrically powered via a battery.
Depots and Loading/Unloading Apparatuses
Compared to major conventional intermodal terminals, freight depots for the proposed system will be smaller and right-sized to enable the prompt transfer of containers between well cars and taxi trucks. The depots will be strategically located. They may consist of a simple open structure with run-through tracks, short sidings, and a loading/unloading apparatus (LULA). Unlike a typical intermodal terminal, where a wheeled jacking-crane lifts containers from a stationary train, a stationary double-girder bridge will support the mobile LULA. Well cars and taxi trucks will be posted by a controlling computer and located to positions beneath the LULA. Large-city freight depots may have multiple LULAs, the number contingent upon the volume the depot is expected to process.The maximum height of a LULA will be slightly greater than the combined height of a well-car floor plus two stacked containers. Lasers will guide taxi trucks to a precise position under the LULA. Additional sensors on the LULA will detect the position of a container on a taxi truck to allow the LULA to raise or lower the container and transfer it horizontally to a well car without the need for a LULA operator.
A provision for temporary storage of well cars may be necessary at some depots to balance supply and demand. Excess empty well cars normally might be stored on short spurs that are adjacent to the depot. Large depots may store empty well cars on a vertical loop-belt elevator that might look like a carpet roll storage machine at a home improvement center.
Some depots will be equipped for repair in place (RIP). When sensors detect a problem in a well car, computers will direct the car to a depot RIP facility where a faulty bogie or other appliance can be quickly replaced with a service-ready counterpart.
Numerous small depots with greatly reduced footprints and extensive automation will allow the economical transport of freight by rail over both long and short distances. Taxi trucks, LULAs, and well cars will guarantee the smooth, continuous flow of containers from shippers to destinations, obviating the need for large regional sorting yards and hump facilities.
Track and Switches
Reliable track and infallible rapid switching are vital to keep the envisioned railroad moving. The use of individual motorized well cars, sans locomotives, that are entering and leaving main and branch lines, freeway style, will necessitate a need for unidirectional running on paired tracks at all but the most isolated and lightly-traveled locations. If all cars are traveling at dedicated speeds, passing and pausing sidings will not be required. In instances where land for double tracking is unavailable, the second track may be elevated over the first. Public lands should also be considered. Corridors, separating freeway lanes, for instance, already exist in many locations. Grade separations at crossings, if not imperative, are certainly desirable.The design of track layouts also should take into account unforeseen hazards, such as severe weather, maintenance requirements, and sporadic localized high usage. While one objective of the railroad is to maintain a continuous flow at high speeds, designs must address real and conceivable safety concerns. For example, longer, more gradual main and branch line exits and entrances than are customary may be warranted.
Switches that snap to position within seconds will minimize the lengths of the slots that are required when single cars are inserted into or separated from a traveling multiple-car platoon. In order for the switches to function with aircraft reliability, the switches should be protected from freezing, dust, and other weather hazards. Inevitably, slowdowns are likely at major hubs, especially when crowded single lines of freight car traffic must be funneled to multiple routes. It may prove expedient to provide steering mechanisms in car bogies to achieve directional changes faster without jolting a container’s contents and to minimize the wear on tracks and wheels.
Energy Sources
Electricity that is generated offsite will power the bogie motors as well as the electric brakes, computers, lights, and horns that are part of each well car. Without electrification, the envisioned economies, as well as the environmental benefits, of an efficient railroad that is constantly in motion would otherwise be impossible to achieve. Offsite stationary generation will encourage competitive sourcing from multiple energy choices including wind, solar, hydro, geo-thermal, natural gas, nuclear fission, and even garbage. As the world proceeds toward a carbon-free environment, the mix of sources ultimately will result in the release of ever smaller quantities of greenhouse gasses. (While acknowledging that large-scale nuclear is currently out of favor, new and safer, small-scale nuclear plants, which are passively cooled and manufactured in factories, could be advantageously spaced along rail corridors and are currently under development. https://www.nuscalepower.com
Most electric power providers offer variable time-of-day pricing. The electricity that is generated will be less costly than the electricity produced by on-board, prime-mover driven alternators. Stationary environmental remediation systems also are characteristically more efficient at removing pollutants than are the apparatuses that are now common on diesel locomotives. Significant further energy savings will be realized through the employment of regenerative braking, and through the elimination of liquid fuel transport and fueling operations that are essential to fossil-fueled, conventional, locomotive operation.
Electrical Transmission
The conduction of electricity to moving trains has traditionally been accomplished by overhead catenary or third rails that either run continuously above or beside a railway track. The current high cost of building and maintaining conventional catenaries is a notable complication to the utilization of offsite stationary power. Moreover, considering height restrictions, pantographs that would be attached above each doublestacked well car on the proposed railroad also would present a significant design challenge.
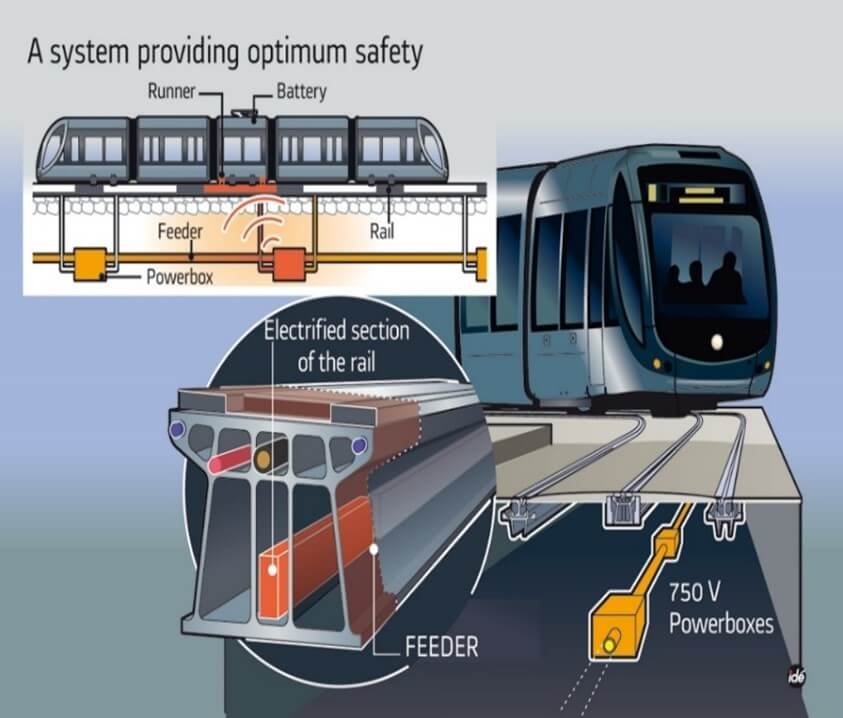
Courtesy, Alsom
Clearly, electrified trains of any type could benefit from new, lower-cost power transmission systems and outside-the-box thinking. Dubai Tram’s, Alstom-built, middle-rail segmented conductor system that is energized only when the pickup shoe of a car passes over an individual segment serves as an example of the type of innovation that is key. Superconductor materials might also yield economies.
Given the advent of battery-power for autos and other roadway vehicles, the notion of a battery-powered well car seems plausible. However, in a foreseeable future, batteries, as a sole source of electrical power for a well car, will likely be incapable of providing the huge quantities of electrical energy that are required for extended rail operations. That being said, smaller batteries installed in each well car feasibly could supply limited energy requirements in local locations, such as central cities, remote customer sidings and branches, and switches and crossings where the placement of track-adjacent electrical transmission feeders would be difficult or impossible. Battery recharging could be accomplished on-the-fly when well cars reenter electrified territory. The economic and advantageous tradeoffs between the added costs of batteries and the savings garnered by eliminating electrical transmission infrastructures at challenging locations should be quantifiable. When battery charging rates and electrical densities are significantly improved over time, electrical conductors and infrastructures could be intermittently spaced further and further apart, thereby reducing the costs of constructing and maintaining continuous railroad electrical power strip or line conductors.
Control
In just over a few recent decades, computers and algorithms have improved productivity, broadened asset utilization, and added capacity to many aspects of railroading, and have streamlined the tasks of scheduling, operation, monitoring, equipment control and maintenance, pricing, customer relations, billing, and financing.
Additional computers on the proposed railroad/transportation network will determine the most economical means of shipping, whether it be by rail, by truck, or by a combination of rail and truck. Dispatching computers will consider the required delivery time; the local cost and availability of fuels and electricity; and the delays that might be encountered due to scheduled maintenance, slow orders, congestion, and adverse weather. Along with GPS and fixed sensors, computers will track shipments, containers, well cars, and taxi trucks, all in real time.
Computers will regulate individual well-car speeds, braking, horn blowing, lighting, and the number of bogie motors that are needed in order to maximize economy while maintaining speed. Computers also will regulate railway traffic to guarantee the orderly movement of individual well cars as the cars enter and leave branches and mains. They will coordinate virtual coupling and platooning of multiple cars and accelerate/decelerate leading and trailing cars that are members of multiple-car platoons to create the slots within the platoons that will allow cars to be smoothly inserted and withdrawn at freight depots and customer sidings.
With signals from drones and other inputs, computers can monitor track conditions and warn of accidents waiting to happen, such as track pull-a-parts and rail fissures. Computers will organize responses and marshal the equipment and personnel that will be required to complete preventive maintenances. Sensors also will monitor aspects of switches, motorized well cars, and taxi trucks. Computers will process the sensor data, schedule maintenance, direct equipment to available repair facilities, and alert an appropriate staff who can ensure that parts and skilled personnel are ready when a compromised well car arrives at a repair facility. Computers and artificial intelligence (AI) will generate and archive reports of maintenance activities, preserve histories, and through algorithms, suggest equipment redesigns or procedural changes that may avoid problems that are associated with occasional and habitual slowdowns or shutdowns.
Computers will process and utilize data from GPS, fixed schedules, rail-side monitors, established protocols, and human controllers. They will provide instant, net-based shipment pricing, and point-to-point pickup and delivery schedules. They will select equipment types, routings, and accommodate special customer needs. Stored data might be used for continuous statistical process control, which in turn can be integrated into new models for individualized customer services and interactions. Billing and payment will be done automatically and immediately.
Computers and algorithms could also assist decision makers and investors by providing an instantaneous accounting of company profitability and by aiding the planning of expansions, curtailments, updates, purchases, and personnel hiring. They will reduce the need for bookkeeping services by automating revenue and expense accounting and inventory tracking. Combined with tax-law provisos, these data could be used to generate tax returns with little help from accounting entities.
Implementation
“How much will it cost?” and “Will it be worth it?” should be among the first questions answered prior to an implementation. Additional questions should include: How many and what types of trucks will be diverted from roads and highways; what new power infrastructures will be needed; how much energy will be conserved; and to what extent will freight transportation’s carbon footprint be reduced? How will foreign shipping be integrated, and to what extent will rail capacity grow? How will controversial aspects of the proposed system be accepted by the public? How will labor be affected? How much and what kind of maintenance will be required? How long will equipment last? What new systems will need to be developed, and how well will they work? What organizations should be included in planning, buildout, and operation?
Further investigations should predict where major system gridlock might occur, identify potential accidents, and suggest alternatives. Other studies should assess what future modifications might be needed. And finally, practical timelines for full implementation should be scheduled.
Equipment R & D and part design can be delegated to specific subsystem teams. Discrete well-car teams, for instance, might be assigned to investigate frames, bogies, sensors, buffers, computer applications, and container attachment and locking mechanisms. Bogie sub-system teams might be further subdivided to investigate bogie frame designs, wheels, split axels, suspensions, motors, and disc brakes. Modeling can assist design.
Equipment can be tested at facilities such as the American Association of Railroads’ (AAR) Transportation Technology Center in Pueblo, CO. To establish an initial proof of concept, pilot plant-scale operational testing might be accomplished in phases on a 100-mile existing branch line that currently services manufacturers, warehouses, and retail outlets. In lieu of an eventual stationary electric network, on-board rechargeable batteries could be utilized as energy sources before investments are committed to a permanent railroad grid. In lieu of a dedicated and eventual container transfer facility (LULA), fork lifts could be used initially to move and load containers.
Railroads have historically implemented many evolutionary and revolutionary changes. The face of railroading has been altered in a constantly evolving process that has spanned more than 180 years. Railroads are now considerably safer, more efficient, more reliable, and more competitive than ever. But, the task is never finished. The next time you are on a freeway, observe the muddle of cars and trucks, think of the chaos it will become in five or ten years, and ponder if the transportation environment we will passing to our sons and daughters is the one we would prefer them to inherit. You will quickly conclude that new solutions to changing transportation paradigms are needed. The writing is on the wall.
* An anecdotal observation to be sure, but then there’s this: 97,988,000,000 (the big-rig interstate truck miles driven in 2018) divided by 525,600 (the minutes in a year) divided by 48,731 (the miles of interstate highways) = ~ 4 (the average number of big- rig trucks on an interstate mile every minute).
https://data.transportation.gov/stories/s/Freight-Transportation-System-Extent-Use/r3vy-npqd
© Wind River Electric Rail